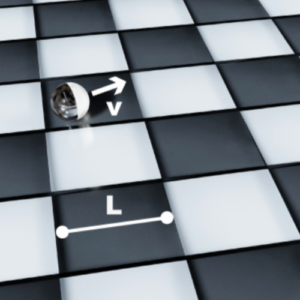
Active Colloids with Position-Dependent Rotational Diffusivity
Miguel Angel Fernandez-Rodriguez, Fabio Grillo, Laura Alvarez, Marco Rathlef, Ivo Buttinoni, Giovanni Volpe & Lucio Isa
Nature Communications 11, 4223 (2020)
doi: 10.1038/s41467-020-17864-4
arXiv: 1911.02291
The non-thermal nature of self-propelling colloids offers new insights into non-equilibrium physics. The central mathematical model to describe their trajectories is active Brownian motion, where a particle moves with a constant speed, while randomly changing direction due to rotational diffusion. While several feedback strategies exist to achieve position-dependent velocity, the possibility of spatial and temporal control over rotational diffusion, which is inherently dictated by thermal fluctuations, remains untapped. Here, we decouple rotational diffusion from thermal fluctuations. Using external magnetic fields and discrete-time feedback loops, we tune the rotational diffusivity of active colloids above and below its thermal value at will and explore a rich range of phenomena including anomalous diffusion, directed transport, and localization. These findings add a new dimension to the control of active matter, with implications for a broad range of disciplines, from optimal transport to smart materials.